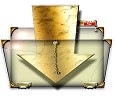
Just before the peak of ascent, runmode 4 engages and we raise the periapsis up to our target altitude to circularize.

Once we push the apoapsis to our target altitude, we cut the engines switch to runmode 3, where we coast up to the apoapsis. This is the slow gentle climb up to our target altitude of 80km. Once we reach a threshold velocity, we transition to runmode 2 where the launch profile maintains a TWR of about 1.25 during the ascent. For example, runmode 1 engages our engines at a safe thrust. As we review the script in detail, you’ll see this represented by the runmode variable. Plummeting head first in a swan dive into the dirt is the exact opposite of our desired outcome, so it’s helpful if we can orient the vehicle regardless of the environment.īecause we’ve decomposed our larger problem into smaller ones, it’s worth noting these represent discrete phases of our launch-and-land objective.
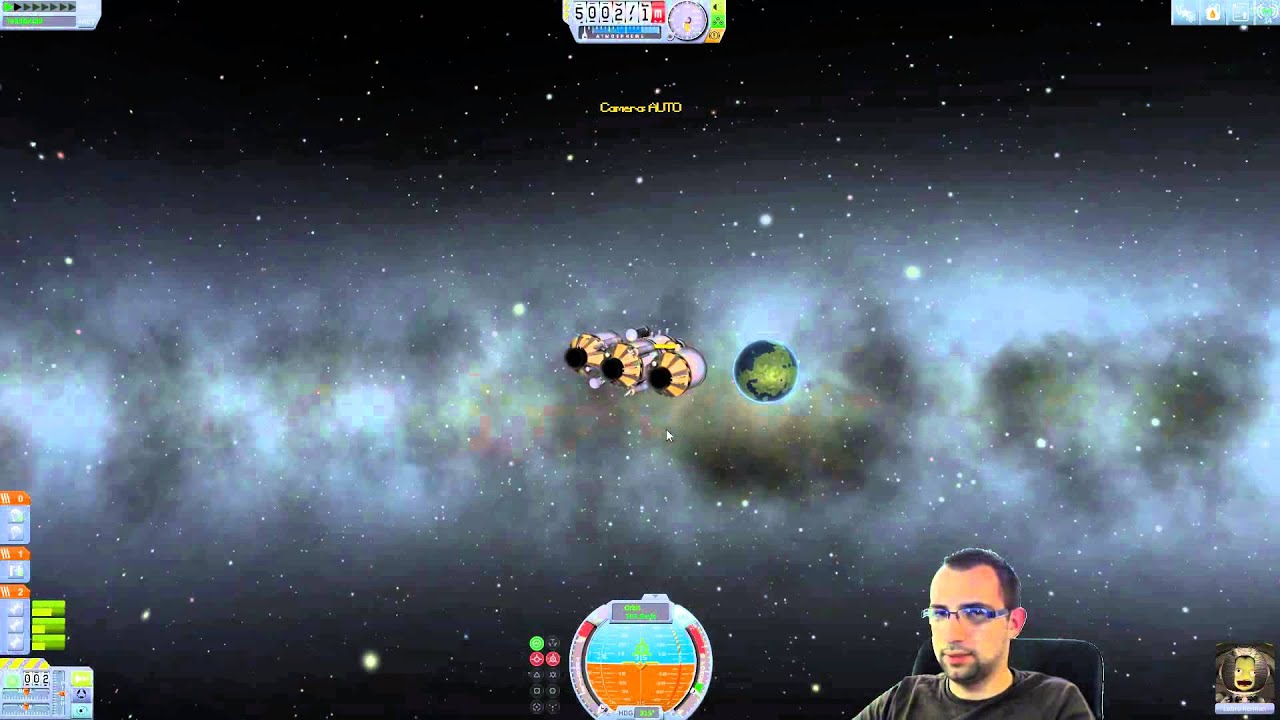
This specific craft has its aerodynamic center co-located with its center of mass to enable atmospheric re-entry with engines pointed retrograde without inverting and impersonating a badminton birdie. This is pretty much as simple as going as fast as we can while staying below transonic speed in the atmosphere. Our first step is to escape the gravity well and stabilize into low orbit. For example, most of the conditions in this experiment use single edge bounded constraints, like “wait until periapsis is greater than threshold.” These simple constraints increase the chance of overall system stability, as they are less sensitive to unexpected side effects. Also, we don’t want to overconstrain the operational parameters, or the system may not converge toward the conditions and be forever stuck in a loop. This means using simple conditions wherever possible. Ideally, each time we wait for a condition to be satisfied, we want the smallest number of components in our logic. But this is about robots, not meat bags, and we’re hoping to make robots who protect the meat bags and deliver them safely to a totally inhospitable environment because that’s what they wanted.īefore we dive into the various phases of the mission plan, let’s establish some rules. Mostly we just turn into frozen desiccated meat bricks if things go horribly wrong, basically the worst freezer burn imaginable. It is worth noting in the vacuum of space there is no organic decay. While it shares its name with the process of death and decaying organic material, that is precisely the outcome we wish to prevent. No pesky staging to interfere with our script.īut enough about rocket science… This is about computer science! :nerd:Īny sensible solution likely involves breaking down the problem into smaller chunks we might be able to tackle individually. This makes it a little easier to design our automation system. The resulting engine has enough thrust to take off from sea level on Eve and enough fuel to accelerate at 1G for a little over a day. Our resident rocket scientist adjusted the aerospike to boost the exhaust velocity up to about 10% of light speed. In the interest of eating the elephant in smaller bites, this exercise uses a vehicle with a modified engine. Normally, it takes a lot of staging and clever redistribution of mass and fuel to coordinate a lunar landing. The good news is aiming high is kinda the point. So, to talk about designing an automated control system capable of landing on Mun is to set the bar high. Moreover, every vessel flies differently hard-learned rules from flight testing with one vessel prove useless or even counterproductive with other vessels. The road to efficient orbital launch is a long punishing ordeal, ripe with uncertainty.
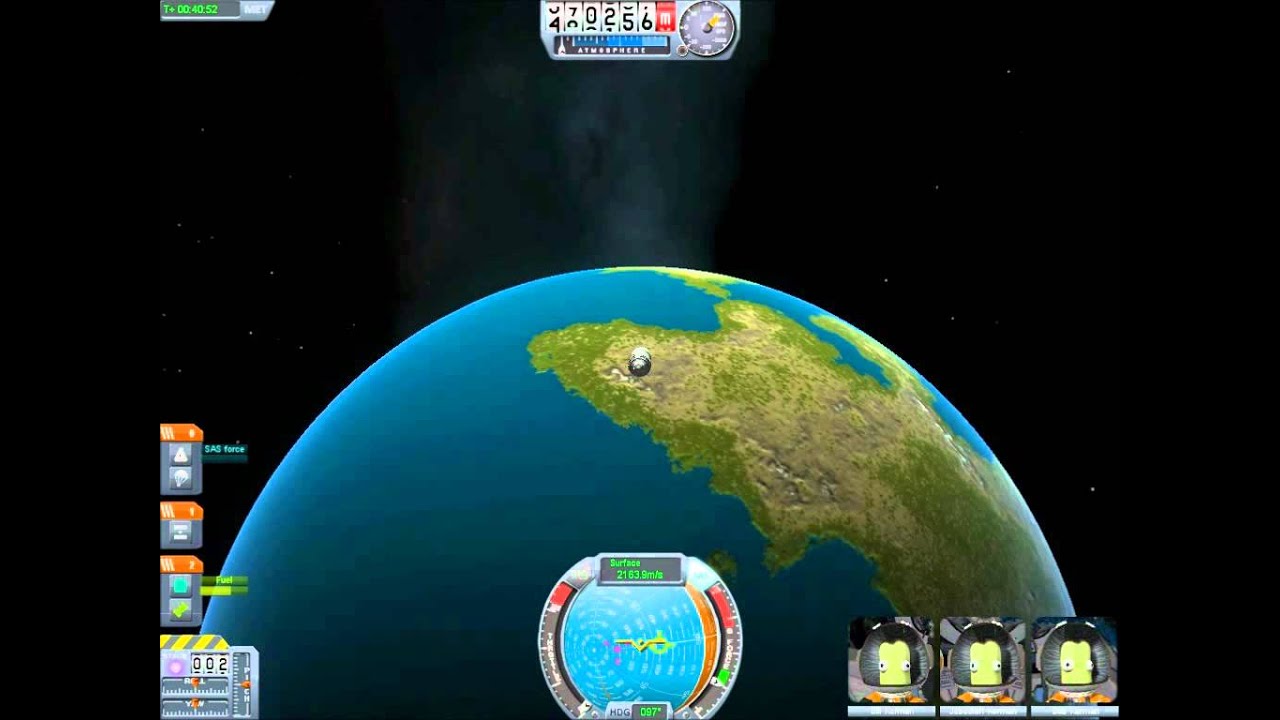
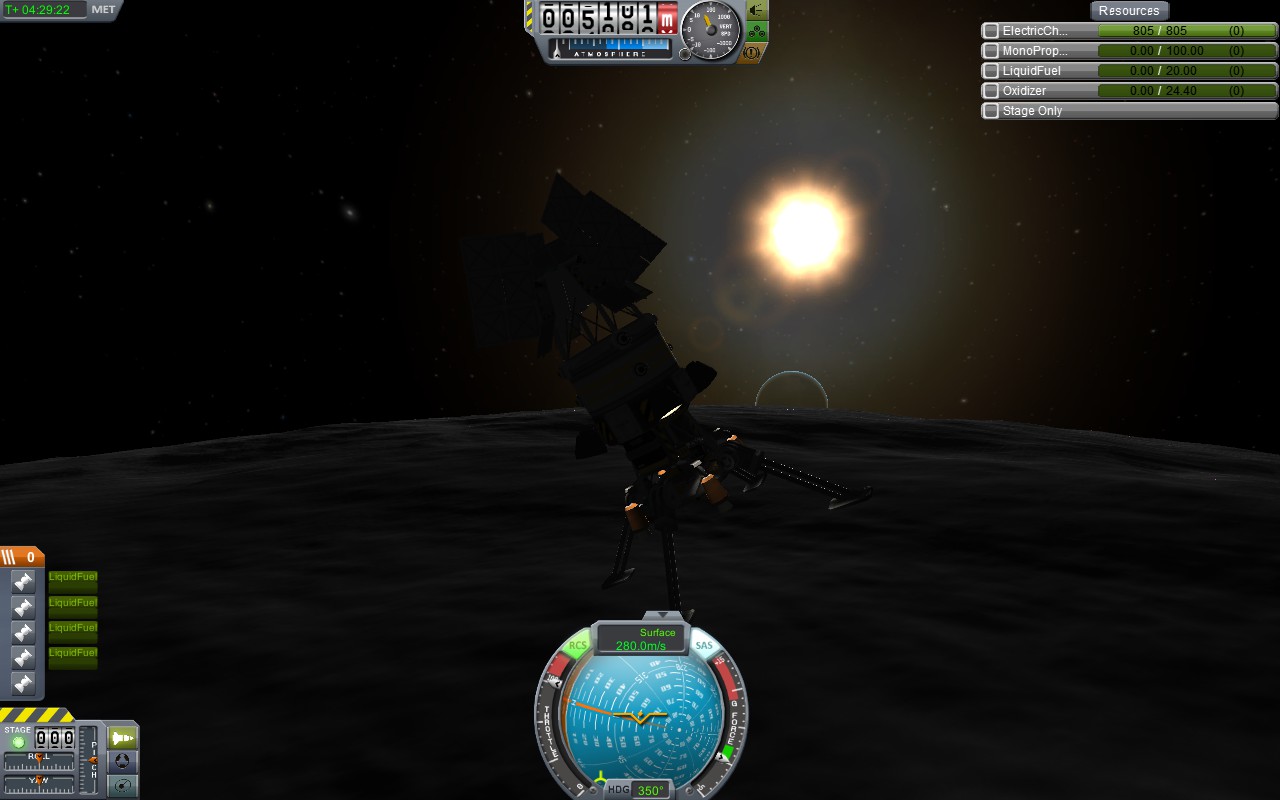
Players often make many mistakes in attempts at suborbital flights before they reach a stable low orbit. Getting to orbit with any vessel in Kerbal Space Program is a challenge that should not be understated.
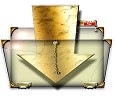